1. Introduction
2. Experimental Procedure
2.1. Seaweed extracts preparation
2.2. TiO2 suspension for sunscreen products preparation
3. Results and Discussion
3.1. Effects of seaweed extract on dispersibility properties of TiO2
3.2. Effects of seaweed extract on sunscreen properties
3.3. Skin patch safety evaluation
4. Conclusion
1. Introduction
The awareness of UV radiation in human skin disorders such as photoaging, erythema, and skin cancer has increased the use of UV protection products. Several products with UV protection have been developed, such as organic and inorganic UV filters that typically apply antioxidants, DNA repair enzymes, and oral protective strategies based on nutritional supplements.1) Sunscreen is an emulsion of water and oil that contains UV filters that can protect the skin from the side effects of UV radiation.2) The demand of sunscreen products raises concerns about the safety and compatibility of these products for both the environment and human health.3) Some studies have confirmed that the presence of organic filters such as benzophenone is highly hazard for both humans and the environment.4,5,6) Compared to organic filters, inorganic filters such as titanium dioxide (TiO2) and zinc oxide (ZnO) are known to be safer and have become promising candidates for UV protection.7) Compared to ZnO, TiO2 as a UV filter is preferable due to its lower solubility in aqueous media, and it is known to be safer compared to ZnO.4,8,9,10) TiO2 nanoparticles (NPs) are widely used in sunscreen production due to their superior properties compared to the bulk state. However, the high surface area of NPs leads to the formation of particle agglomeration and disruption of nanoparticle properties into bulk materials. Therefore, dispersing agent is highly required to prevent the particles from forming agglomeration.
Dispersing agent is a substance that is added to a suspension of solids in a liquid media to improve the separation between particles and prevent the agglomeration formation. In sunscreen products, Stearic acid is often used as a dispersing agent.11) However, according to the MSDS data sheet of stearic acid, it is noted that stearic acid is highly hazardous to aquatic life and may cause skin irritation in humans.12) Therefore, to overcome this problem, we have tried to study and develop a natural-based dispersing agent by utilizing the natural ingredients of Korean sea mustard. Due to the natural based ingredients of this dispersing agent, it is expected to be safe for humans and the environment. Sea mustard seaweed or Undaria pinnatifida is a kind of brown seaweed largely found in South Korea, where it is called Miyeok. Globally, seaweeds are used for human food, animal fodder, chicken, and aqua feed.13) In Korea, seaweed, especially Miyeok, is often used as a food source and as a moisturizing agent in cosmetics. However, to date, according to our knowledge, the use of seaweed extract as a dispersing agent has been not published yet. Several types of seaweed such as Undaria pinnatifida contain an interesting group of complex polysaccharides called phycocolloids such as agar, alginates, and carrageenan as shown in Fig. 1.13,14) Among these polysaccharides as shown by Fig. 1, alginates that contain carboxyl groups can be utilized as a good candidate to stabilize metal oxide such as TiO2. According to the previous study, a material or substances can be utilized as a dispersing agent due to the presence of moiety such as amine and carboxyl thus can easily absorb into the particle’s surfaces. It was found that carboxyl moiety turns out to be the most effective one because it interacts strongly with the base sides that are often present on the metal oxide surfaces.15)
Therefore, considering the safety concerns for humans, the environment, and the presence of alginates in seaweed extract brings a great reason for us to utilize seaweed extract as a natural dispersing agent to improve the stability of the TiO2 particles in the dispersing medium.
In this study to improve the stability of TiO2 nanoparticles, the concentration of seaweed extract as a dispersing agent has been studied. It was found that 3 wt% of seaweed extract based on TiO2 concentration was the optimum concentration to be able to disperse TiO2 NPs. Further, the effects of seaweed extract as a dispersing agent on TiO2 stability and sunscreen properties have been studied.
2. Experimental Procedure
2.1. Seaweed extracts preparation
Well-dispersed TiO2 nanoparticles were produced through surface modification with surface modifiers extracted from seaweed in an aqueous medium. The surface modifiers were extracted from seaweed using hot water with ultrasound assistance. Seaweed extract was obtained from fresh miyeok seaweed purchased from a local farm in Kijang, South Korea. The extraction process took place in a 250 ml four-necked round-bottom flask equipped with a reflux condenser and rubber cap. The purchased fresh seaweed was thoroughly washed with tap water to remove dirt and any substances carried in from the ocean. Subsequently, the seaweed was rinsed continuously with distilled water, dried, and ground into powder. The ground seaweed powder was weighed and mixed with distilled water at a powder-to-solvent ratio of 1:20 (w/v) in a glass beaker. The seaweed-water solution was then subjected to ultrasonication at 20 kHz with an amplitude level of 60 % for 15 minutes. The ultrasound facilitated greater solvent penetration into the samples by increasing the contact surfaces between the solid and liquid phases, allowing the solute to quickly diffuse from the solid state to the solvent. The solution was transferred into the round-bottom flask and placed in an oil bath preheated to 70 °C. The extraction process was carried out for 30 minutes and cooled to room temperature. The seaweed extract was collected by centrifugation, precipitated overnight in ethanol at 4 °C, filtered, and vacuum-dried at room temperature. The obtained dried extract was stored in a refrigerator and is now ready for use as a surface modifiers to stabilize TiO2 nanoparticles.
2.2. TiO2 suspension for sunscreen products preparation
To investigate the effectiveness of seaweed extract as a surface modifiers, a mixture solution of TiO2 and prepared seaweed extracts in an aqueous suspension was charged in a glass beaker. Distilled water and 3 wt% of seaweed extract were charged into a glass beaker and stirred with a magnetic bar for a specified duration to achieve a well-mixed solution. To this solution, 40 wt% of TiO2 powder was added, stirred for a designated period, and subjected to ultrasonication at 20 kHz with an amplitude level of 60 % for 30 minutes to disperse the initially agglomerated TiO2 nanoparticles. The well-dispersed TiO2 suspension, achieved by combining TiO2 nanoparticles with seaweed extract as surface modifiers through ultrasonication methods, was utilized as an active ingredient for sunscreen products development. Two types of sunscreens, aiming to achieve a sun protection factor (SPF) of 35, were prepared, one from the suspension of bare TiO2 and the other from TiO2 modified with seaweed extract. The sunscreens were formulated at KB Cosmetics by blending the TiO2 suspension with other sunscreen ingredients, including glycerin, sorbitol, behenyl alcohol, and fragrance.
The distinction between the two sunscreens lies in the addition of a commercial dispersing agent to the sunscreen prepared with bare TiO2 suspension, while no additional dispersing agent was introduced into the sunscreen prepared with TiO2 modified with seaweed extract. The capability of seaweed extract to modifiy TiO2 nanoparticles was investigated using FT-IR spectroscopy to confirm the adsorption of seaweed extracts on the TiO2 particle surfaces. The stability and dispersion properties of the TiO2 suspension, influenced by the addition of seaweed extract, were affirmed using a particle size analyzer (PSA) and sedimentation test rate through the application of centrifugal forces. Conclusively, the sunscreen properties were analyzed by calculating the Sun Protection Factor, and skin brightness value before and after the sunscreen was applied to the skin.
3. Results and Discussion
3.1. Effects of seaweed extract on dispersibility properties of TiO2
The adsorption of seaweed extracts onto TiO2 surfaces was confirmed by FTIR analysis. As shown in Fig. 2, the bare TiO2 has three bands, which is in good agreement with the literature. The first broadest band, at 3,438 cm-1, indicates the stretching vibration of hydroxyl group OH of the TiO2.16) The second band, at 1,629 cm-1, indicates the bending modes of water Ti-OH.17) The broad band in the 1,000~500 cm-1 region, belongs to Ti-stretching and Ti-O-Ti bridging stretching modes.18) Further, the second spectra in Fig. 2 as described in the black spectrum, which belongs to the seaweed extract, shows several peaks as described below. The broad peak at 3,430 cm-1 indicates the OH groups. The peaks around 2,923 cm-1 indicate the C-H stretching of CH2. The strong peaks at 1,631 cm-1 can be attributed to the C=O vibration stretching of the carboxyl group.19) The presence of a peak around 1,053 cm-1 can indicate C-O stretching, which indicates the presence of uronic acids such as guluronic or mannuronic acid.20,21) Furthermore, the third spectra in Fig. 2 as described by the blue color spectrum, which belongs to TiO2 which contains seaweed extract shows the presence of several new peaks compared to bare TiO2 at 1,533 cm-1, 1,403 cm-1, and 1,045 cm-1 indicating the asymmetric and symmetric stretching bands of COO- respectively due to the splitting of the carboxylates group with TiO2 surfaces. The presence of the new peak in the FTIR spectra of TiO2 which contains seaweed extract indicates that seaweed extract has been well adsorbed onto the TiO2 surface by carboxyl anchoring mode which is expected to belong to alginate as shown in Fig. 2(b).22)
To understand the agglomeration and the dispersion stability of particles in the suspension media, particle sizing approaches such as dynamic light and static scattering to infer the stability of the colloidal suspension have been used a lot in previous studies. However, this method is only useful for observing the kinetics of the early stages of agglomeration. Therefore, the colloidal stability would be better characterized if both particle size distribution and the transient nature of the suspension could be determined; thus, it can be done by studying the sedimentation behavior through gravitational or centrifugal forces.23) In this study to characterize the TiO2 suspension stability, particle size distribution and the transient nature of TiO2 suspension were examined by particle size analysis and sedimentation test rate by centrifugal forces. Further, the particle size distribution width was calculated based on the span of the volume-based size distribution as defined as:
where, D90 is the particle diameter for the 90th cumulative mass, D10 is the particle diameter for the 10th cumulative mass, and D50 is the particle diameter for the 50th cumulative mass. A higher value of the span indicates a wider particle size distribution.
To determine the transient nature of TiO2 suspension, centrifugal forces were applied and TiO2 suspension stability was monitored by the sedimentation behavior. For stable colloidal suspension, the particles must be not dependent on the centrifugal forces, which means that particles should remain uniformly dispersed and no significant sedimentation should be observed. However, for unstable suspension, the particle must be dependent on the centrifugal forces due to the inability the particles to repulse each other thus resulting in agglomeration formation and forcing the particle to settle down into the bottom of the centrifuge tube.
As shown in Fig. 3. Before centrifuged, the suspension prepared from bare TiO2 shows particles clump on the centrifuge tube’s walls indicating the presence of the initial agglomeration. Further, after being centrifuged the presence of centrifuge forces brings the particles to come and connect each other to form agglomeration and finally settle down on the centrifuge tube’s bottom. However, for the suspension prepared from TiO2 with seaweed extract addition shows no clumps formation on the centrifuge tube indicating that the suspension is agglomeration-free and being well dispersed in the suspension. Even after being centrifuged, there is no sediment being observed on the centrifuge tube indicating that these particles are not dependent on the centrifuge forces. The independence of these particles from the centrifuge forces indicates that these particles are being well stabilized which is expected due to the adsorption of seaweed extract through the carboxylate moieties on the TiO2 surfaces as confirmed by FTIR. This adsorption of carboxylates of seaweed extract compounds on the TiO2 surfaces can form an organic layer on the TiO2 surface and generate repulsive forces between particles to prevent the agglomeration formation and result into well well-stabilized TiO2 suspension.
Further to confirm the effects of seaweed extract adsorption on the stability of the TiO2, the particle distribution and span value have been calculated according to Eq. (1). It was known that the primary size of the TiO2 nanoparticles was 15 nm; however, as confirmed by particle size analyzer, the particle size of bare TiO2 nanoparticles in aqueous suspension shows a large number of D50:0.741 um. This large particle size is assumed due to the inability of particles to overcome the Van Der Waals attraction forces thus bringing the particles together and colliding to form agglomeration. However, as seaweed extract was added to TiO2, the particle size changed to an extremely low number with D50:044 um, as confirmed in Fig. 4. The presence of an organic layer of seaweed extracts on the TiO2 surfaces can generate repulsive forces between particles due to steric hindrance thus preventing the particles from colliding with each other. Therefore, agglomeration formation can be prevented. Accordingly, smaller particle sizes can be observed as shown by the shifting of the peak into the lower value as shown in Fig. 4(a). Furthermore, by substituting the D90, D50, and D10 which were obtained from the particle size analyzer into Eq. (1), the span values can be calculated as shown in Fig. 4(b). Through Fig. 4(b), it was clearly seen that the span value of the TiO2 thus stabilized with seaweed extract has a lower value of 1.8 compared to 2.2 for bare TiO2 suspension indicating the lower size distribution of TiO2 suspension which stabilized with seaweed extract.
3.2. Effects of seaweed extract on sunscreen properties
TiO2 is known as an effective UV ray-filtering material to protect the skin from UV ray damage from sunlight. UV rays are initially split into three parts: UVA (315~400 nm), UVB (280~315 nm), and UVC (100~280 nm). Since UVC has the shortest wavelength, its penetration into the skin is often neglected, because UVC is almost absorbed by stratospheric molecular oxygen to produce ozone. However, UVB and UVA, which have longer wavelengths, are known to penetrate deeper into the skin and cause health problems such as skin damage and even cancer.24,25) From the literature study, it is well known that the presence of TiO2 in sunscreen products can protect human skin from the absorption of UV rays and block them from penetrating the skin.26)
In this study, two types of sunscreen that are supposed to have sun protection factor (SPF) 35 were prepared from the suspension of bare TiO2 and TiO2 stabilized with seaweed extract. The two sunscreens were produced by mixing the TiO2 suspension with some sunscreen ingredients such as glycerin, sorbitol, and behenyl alcohol and fragrance. The difference between the two sunscreens lies in the addition of a commercial dispersing agent into the sunscreen which is prepared with bare TiO2 suspension, and there is no additional dispersing agent was added into the sunscreen prepared with TiO2 stabilized with seaweed extract suspension.
In accordance to calculate the Sun Protection Factor (SPF) of sunscreen products, a laboratory test that reflects how well the products protect the skin from UV(B) radiation has been done by using an artificial ultraviolet radiator with a 300-watt xenon lamp as a light source. SPF measurements were done by using “Measurement Standards for UV Protection Efficacy” in accordance with the Ministry of Food and Drug Safety Notice No. 2019-47 in accordance with Article 4 of the Cosmetics Act. The SPF value was determined by calculating how much the amount of UVB radiation is required to cause sunburn on skin protected by sunscreen, divided by the amount of UV(B) required to cause sunburn on unprotected skin.
Through Fig. 5(a) it can be confirmed that sunscreen formulated with TiO2 stabilized with seaweed extract has higher adsorption of UVA and UVB compared to sunscreen formulated with bare TiO2. The more the TiO2 absorbs the UV rays, the less the light is transmitted through the sunscreen, and the less the UV radiation penetrates the skin.26) According to the previous study, the SPF and UV adsorption value of sunscreen products have a relative correlation, the higher the UV adsorption, the higher the SPF value.27) As shown by Fig. 5(b), the UV adsorption and SPF value show a correlative relation, which is in good agreement with the literature study.27)
As shown by Fig. 5, The SPF value of the sunscreen products with human application test on 10 subjects with skin types of I, II, and III were selected according to the methods and standards for measuring UV protection effects. It was found that sunscreen formulated with TiO2 stabilized with seaweed extract has an SPF value of 36, and sunscreen formulated with TiO2 with the addition of a commercial dispersing agent failed to reach SPF 35 and fall into a lower value of SPF 34. A higher SPF value of the sunscreen formulated with TiO2-containing seaweed extract compared to the sunscreen formulated with bare TiO2 can be obtained due to the better dispersion properties, smaller particle size as well and narrow size distribution as well. The higher dispersibility properties of TiO2 in sunscreen formulated with TiO2 stabilized with seaweed extract will give affection to the spreading properties of sunscreen when it is applied on the skin surfaces. Better dispersion properties, smaller particle size, and narrow size distribution will reduce the void space between the TiO2 particles when applied to the skin. This will improve the sunscreen coverage on the skin and improve the sun protection as well, as shown by the higher SPF value. On the other hand, the sunscreen formulated with bare TiO2 showed that the SPF value failed to reach 35 and fell into SPF 34 which supposed as the effect of initial agglomeration which has been formed in the suspension before being used to formulate the sunscreen products.
The presence of the TiO2 agglomeration in the initial suspension could lead to the formation of hard agglomeration which is difficult to re-agglomerate despite the addition of addition the commercial dispersing agent during sunscreen production. Furthermore, the effects of the agglomerated particles and wider size distribution of the particles will leave more void between particles when it is applied to the skin. The more voids on the skin, the more UV rays penetrate into the skin, as a result, sun protection properties will be disrupted as shown by the lower SPF values in Fig. 5(b).
Further, the effects of dispersion properties of TiO2 suspension on the white opaque appearance of sunscreen products when it is applied on the skin surfaces have been studied. According to the literature study, it was claimed that the presence of TiO2 can result in a white opaque appearance on the skin as sunscreen is applied, which is not preferred for cosmetics.28) Therefore, to overcome this problem, some formulation strategies such as dispersion can be applied. In this study, the effects of TiO2 dispersion stability to overcome the white opaque color of sunscreen products were confirmed by analyzing the skin brightness value before and after sunscreen was applied by using VISIA-CR and spectrometer CM-2600D, Minolta, Japan.
According to the VISIA-CR image as shown in Fig. 6(a), before the sunscreen was applied, both countenances showed a clear image in standard and UV-NF modes. However, when sunscreen is applied, the countenances show a white appearance in standard mode and a black appearance in UV-NF modes, indicating that sunscreen has been applied to the skin. Further, to examine the effects of TiO2 dispersion properties in the sunscreen products on the skin appearance, the skin color changes before and after sunscreen was applied to the skin were measured by using a color difference meter (Spectrophotometer CM-2600D, Minolta, Japan) as shown in Fig. 6(b).
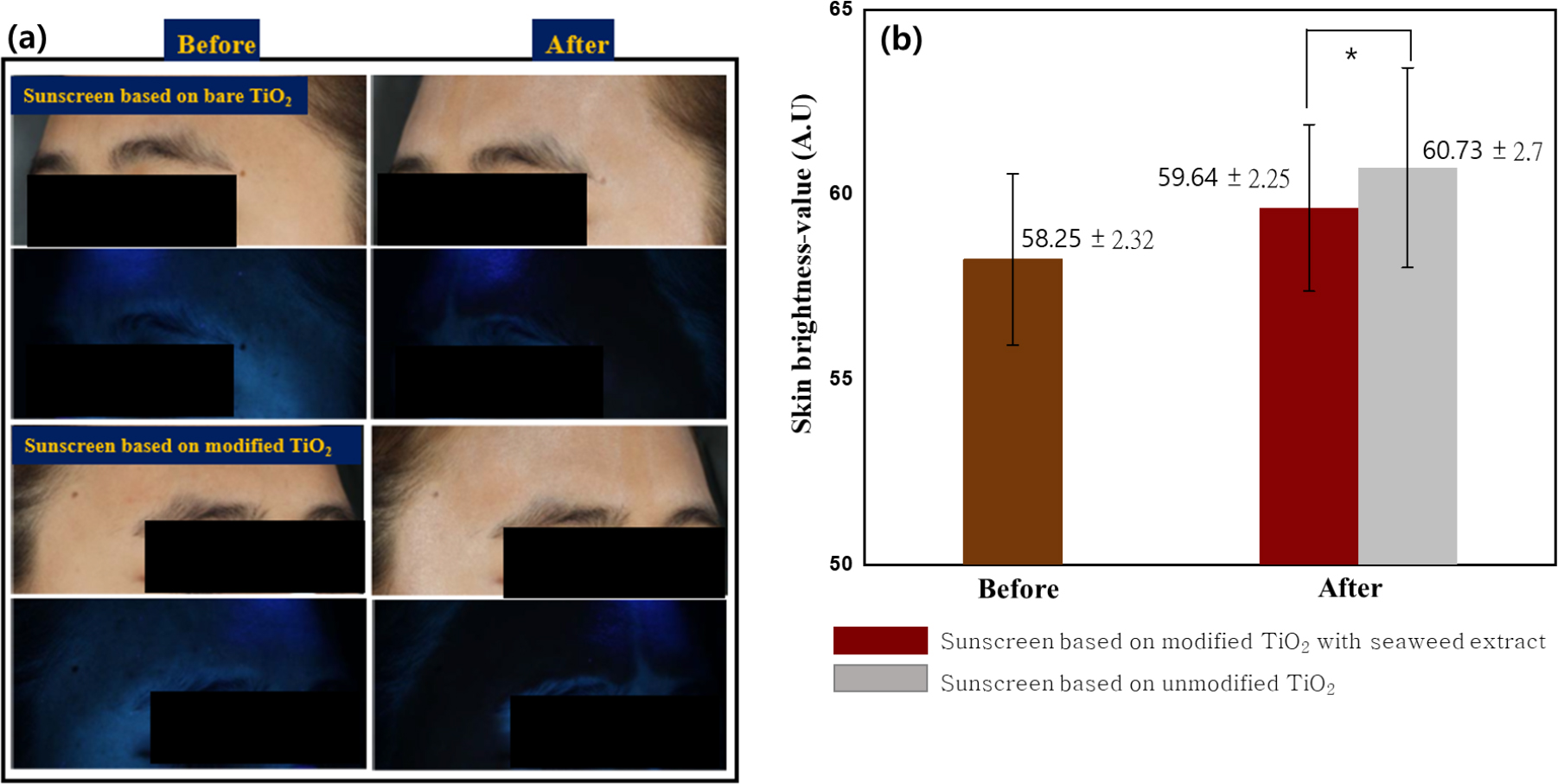
Fig. 6.
Skin brightness value of TiO2 (a) obtained from VISIA - CR image (a.1) sunscreen based on bare TiO2 in standard (top image) and UV-NF mode (bottom image), (a.2) sunscreen based on TiO2 containing seaweed extract in standard (top image) and UV-NF mode (bottom image), (b) Spectrophotometer CM-2600D, Minolta, Japan. *: p < 0.05 (0.003) (p-value by Independent t-test).
For statical analysis, the results of the skin color change for the area after sunscreen was applied on the skin were analyzed by using the independent t-test methods. All the statistical results were considered to have statistical significance change when the significant difference was 5 % (p < 0.05), which is the most commonly used difference in biological statistical analysis, and SPSS 18.0 software was used for statistical analysis. According to the results as shown by Fig. 6(b), bare skin has a brightness value of 58.12. However, when the sunscreen is applied on the skin, the skin brightness value changes to 60.73 for sunscreen formulated with bare TiO2 and to 59.64 for sunscreen formulated with TiO2 modified with seaweed extract, respectively. It was found that there is a statistically significant difference in skin color after using sunscreen formulated with modified TiO2 with seaweed extract compared to sunscreen formulated with unmodified TiO2 (p < 0.05).
The higher change of the skin’s brightness value when the sunscreen formulated with bare TiO2 is applied on the skin indicated that the higher white opaque color has resulted, which is undesirable for cosmetics application as mentioned above.28) This undesired result of a higher white opaque color on the skin is assumed to be the consequence of the lack of TiO2 suspension stability. The lack the suspension stability of TiO2 and the higher agglomeration results make into the increase of the particle size and as a consequence, a higher white opaque color on the skin is shown for the sunscreen formulated with bare TiO2 when applied on the skin. However, on the other side, when the sunscreen formulated with TiO2 stabilized with seaweed extract is applied on the skin, it can be seen that change in the skin brightness value is minimized. This indicates that the skin color does not change much, which means that white opaque color is less detected. These results can be obtained due to the presence of seaweed extract in TiO2 suspension thus providing stabilization and lessening the agglomeration. As a more transparent appearance and less white opaque color of the skin can be obtained as shown in Fig. 6.
3.3. Skin patch safety evaluation
In addition, to evaluate the irritation effects on the human skin, sunscreen patch testing was tested on 30 females with ages ranging about 21 to 54 years old. To make sure the measurement condition is the same, the test site was kept clean and dry, and the skin was stabilized at a constant temperature and humidity (22 ± 2 °C, R.H 40~60) for at least 30 minutes before proceeding. Furthermore, the skin is wiped with 70 % ethanol and drying. Further, the sunscreen was dripped into the Finn chamber and placed on the backside of the subjects. The patch was removed after 24 h and the test site was marked with a marking pen. Further, the test site is observed after 30 minutes, 24 h, and 48 h. The skin reaction was evaluated according to the criteria of the International Contact Dermatitis Research Group (ICDRG) as shown in Table 1 and confirmed that there are no reactions were found (Supplementary Table 1).
Table 1.
Scoring of patch test reactions according to ICDRG recommendations.29)
4. Conclusion
As confirmed by this study, it can be seen that seaweed extract can be used as a good dispersing agent for TiO2 NPs. According to the FTIR, it was confirmed the stabilization of TiO2 was improved due to the presence of carboxyl groups that adsorbed on TiO2 surfaces which are expected to belong to the alginate substance present in seaweed extract. Due to this adsorption, an organic layer is present on the TiO2 surfaces and hindering the TiO2 particles from forming agglomeration as a result a better dispersion properties can be obtained as shown by the smaller particle size, and narrowing the size distribution. Furthermore, as the stabilized TiO2 is used as an active agent in sunscreen products, better sunscreen properties such as higher UV protection, better-preading properties, and less white opaque color on the skin can be obtained. Also, the natural properties of seaweed extract can give more advantages to human skin and environmental safety. It was confirmed that there is no negative reaction to the human skin, indicating that the product is a non-irritating product as confirmed by the ICDRG patch test. From the results of this study, we are expecting that there will be an increase in the utilization of seaweed in the cosmetics sector, especially as a dispersing agent which is expected to increase the commercial value of the “Miyeok” itself. However, a further study of seaweed extract compound analyzation is maybe required, to make it to be more applicable for dispersing agent fabrication.